Revealing the brain’s mysteries, one neuron at a time
Eric Olson | April 18, 2019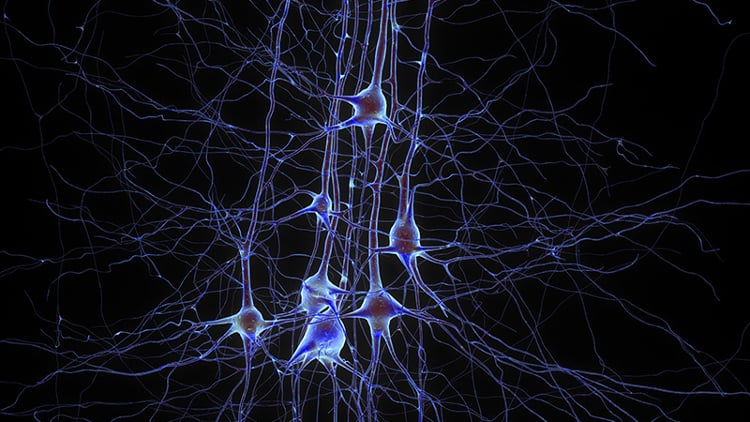
As the seat of consciousness, the brain makes humans different from all other living creatures. Although it plays a central role in defining humanity, a precise understanding of how the brain functions still eludes researchers.
Certain regions of the brain light up during certain activities – for example, the occipital lobe (one of the four major lobes of the cerebral cortex) serves as the brain's visual processor – and science has an appreciation of the anatomy and function of individual neurons.
In many ways, however, the brain remains an enigma, including exactly how the brain turns thoughts into actions or stores and retrieves memories.
To understand how the brain works, scientists study it not only at the level of individual cells and their connections to each other – neurons and synapses – but also at the level of the entire organ in an attempt to account for its massive number of interacting cells. This is no easy task, as the brain is made up of some 100 billion neurons connected by 100 trillion synapses.
The Blue Brain Cell Atlas plots the densities and positions of all neurons and glia in the brain of a mouse. This image maps the more than 22 million neurons that make up the cerebral cortex. Source: Blue Brain Project. (Click image to enlarge.)
Efforts over more than a decade, however, have paved the way to a better understanding of the intricacies of the brain’s operation.
In 2013, the United States announced the Brain Research Through Advancing Innovative Neurotechnologies (BRAIN) Initiative. That same year, the European Union kicked off its Human Brain Project. In 2014, Japan launched the Brain Mapping by Integrated Neurotechnologies for Disease Studies (Brain/MINDS) project. China’s 15-year Brain Project was given the go-ahead in 2016.
Switzerland’s Blue Brain Project, founded in 2005, predates these initiatives. Blue Brain seeks to build a fully simulated digital reconstruction of the human brain. On the path to building a complete human brain model, the project has paved the way with a replica of the small brain of the mouse.
In November 2018, the project released a digital 3D atlas of a mouse brain, showing for the first time the numbers, locations and types of each of the over 70 million neurons in the mouse’s 737 brain regions.
Higher dimensions
A digital replica of a portion of the neocortex (left). An artist’s attempt to illustrate the higher-dimensional cliques and cavities that form as neurons respond to stimuli (right). Source: Blue Brain Project
Scientists funded by the Blue Brain project are also making strides in visualizing the patterns of neuron activation that occur as the brain processes information.
To accomplish this, they simulated a section of the neocortex as a network of connected nerve cells: so-called “pyramidal” neurons, the fundamental computational elements of the neocortex. Each individual neuron was modeled with its incoming and outgoing connections, taking into account the directionality of synaptic transmission in which each neuron transmits information along certain paths.
When stimulated, neurons formed functional cliques, linked groups of neurons pulsing with correlated activity. These cliques were arranged in shapes that can be thought of as geometric objects that surround holes that the researchers call cavities. The scientists analyzed the networked structure of cliques and cavities with a mathematical technique known as algebraic topology.
Remarkably, the researchers found that as neurons respond to a stimulus, the geometric shapes defined by the cliques and cavities evolve into structures with higher dimensions than the 3D world we are familiar with.
“The progression of activity through the brain resembles a multi-dimensional sandcastle that materializes out of the sand and then disintegrates,” said Mathematician Ran Levi of the University of Aberdeen.
The researchers confirmed their simulated findings by carrying out experiments on rat neurons. To do that, they set up patch clamp probes to measure the electrical activity of up to 12 neurons at a time, then observed behavior similar to that found in simulations. What they found was that the real brain tissue exhibited an even greater number of cliques than was demonstrated in simulation. The researchers theorize that this is because they did not model the brain's plastic rewiring that occurs over time as an organism develops and learns, which increases organizational complexity.
Human uniqueness
Researchers reconstructed digital models of human layer 3 (L3) dendritic spines. (A), (C) and (E) are confocal microscopy images of an actual human L3 pyramidal neuron. (B), (D) and (F) show the digital reconstructions. (G) and (H) display manual measurements of neural neck diameters and spine head areas. Source: Eyal, Verhoog, Testa-Silva, Deitcher, Benavides-Piccione, DeFelipe, de Kock, Mansvelder and Segev. (Click image to enlarge.)
Even tissue from a rat’s brain, however, cannot reveal the human brain's full sophistication. For that, scientists carrying out a different study, building a virtual 3D model of pyramidal neurons in the neocortex with properties defined by observations obtained in biopsies of human neurons.
Differences between the neocortices of humans and rodents have been known from previous research. For example, the thicker human neocortex has a thousand times more neurons than the rat neocortex. In addition, compared to their rodent counterparts, human pyramidal neurons are physically larger, contain more branches that receive three times as many synapses – 30,000 – and feature smaller specific capacitances that facilitate easy production and transmission of electrical pulses.
The new computer simulation of human pyramidal neurons revealed new details about just how exceptional the brain cells of humans are compared to those of rodents.
“Human neurons are… fundamentally more powerful in their ability to integrate, process and store information,” said Professor Idan Segev of the Hebrew University of Jerusalem, Israel.
Human pyramidal neurons receive three times as many synapses as those of rats. They also can handle almost twice as many pulses of voltage traveling from the dendrite to the cell body – so-called local dendritic spikes – at the same time.
Moreover, unlike rat neurons, human neurons provide more than a basic linear sum of the electrical signals they receive. Instead, human neurons non-linearly integrate signals in branches of their dendrites (a short branched extension of a nerve cell, along which impulses received from other cells at synapses are transmitted to the cell body) before summing them up to produce an output at the axon – akin to a two-layer neural network. By comparison, rodent neurons are limited to the signal-processing capabilities of a one-layer neural network. As a result, human pyramidal neurons can store almost four times more information as their rodent equivalents.
Full-brain simulation
Studies like these are shedding light on the brain’s function. But to truly understand how it works, a complete simulation of all of the brain’s billions of neurons and trillions of synapses is needed. To date, the most advanced brain simulation software running on the fastest supercomputers is only capable of simulating about 1% of the brain.
Each of SpiNNaker’s Spinn-5 circuit boards contains 48 chips with 18 processing cores per chip. Source: IEEE International Joint Conference on Neural Networks
Instead of modeling the brain in software running on traditional supercomputers, which lack the power to fully simulate the brain, some scientists are developing neuromorphic computers. These machine's architectural design takes their inspiration from biological brains.
Researchers funded by the Human Brain Project are advancing a neuromorphic computer called SpiNNaker (Spiking Neural Network Architecture), made up of more than a half-million ARM processing cores (a family of reduced instruction set computing architectures for computer processors) contained on 600 circuit boards.
In a study, scientists put to work 1% of the machine’s total capacity to model the full density of cells in 1 mm2 of cortex – about 80,000 neurons and 300 million synapses – with similar speed and power efficiency as a software simulator known as Neural Simulation Tool.
[Discover ARM Microprocessor Chips on Engineering360.]
In the future, optimized instruction sets and scaled-up hardware could allow SpiNNaker to come closer to a full simulation of the human brain than ever before.
Even if SpiNNaker eventually is able to represent every one of the brain's neurons and synapses, the simulation will need to advance beyond its simplified point-neuron model with static spiking currents to take into account complexities such as the effects of conductance and plasticity.
For now, a full picture of the brain’s operation – and, thus, of humans – remains locked in complexity. Researchers are hard at work, steadily breaking down that barrier to reveal the brain’s mysteries.
Further reading
Reimann MW, Nolte M, Scolamiero M, Turner K, Perin R, Chindemi G, Dłotko P, Levi R, Hess K and Markram H (2017) Cliques of Neurons Bound into Cavities Provide a Missing Link between Structure and Function. Front. Comput. Neurosci. 11:48. doi: 10.3389/fncom.2017.00048
Eyal G, Verhoog MB, Testa-Silva G, Deitcher Y, Benavides-Piccione R, DeFelipe J, de Kock CPJ, Mansvelder HD and Segev I (2018) Human Cortical Pyramidal Neurons: From Spines to Spikes via Models. Front. Cell. Neurosci. 12:181. doi: 10.3389/fncel.2018.00181
van Albada SJ, Rowley AG, Senk J, Hopkins M, Schmidt M, Stokes AB, Lester DR, Diesmann M and Furber SB (2018) Performance Comparison of the Digital Neuromorphic Hardware SpiNNaker and the Neural Network Simulation Software NEST for a Full-Scale Cortical Microcircuit Model. Front. Neurosci. 12:291. doi: 10.3389/fnins.2018.00291